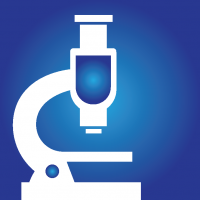
By: Reanne Li
Original Article: Ko, J., Mohtaram, N.K., Ahmed, F., Montgomery, A., Carlson, M., Lee, P.C.D., Willerth, S. M., Jun, M.B.G. (2013). Fabrication of poly (e-caprolactone) microfiber scaffolds with varying topography and mechanical properties for stem cell-based tissue engineering applications. Journal of Biomaterials Science, Polymer Edition, 25(1), 1-17. Find original article here.
Introduction
Tissue engineering is a rapidly evolving field. It is involved in the repair and replacement of tissues, including bone, cartilage, skin and nerve. Recently, tissue engineers have been developing biomaterial scaffolds to mimic the microenvironment of a specific tissue type. The idea is that if the microenvironment is synthetically replicated, coupled with embryonic stem cells (ESCs), and delivered to the damaged tissues, the scaffold will induce the cells to differentiate into the desired cell type, which will hopefully lead to the regeneration of the target tissue.
Among the many techniques that researchers employ to make biomaterial scaffolds, electrospinning stands out for its controllability and ability to include drugs inside the fibers that constitute the scaffold. Two different methods of electrospinning include:
- Solution electrospinning involves dissolving a polymer into a solution, and applying a high voltage to induce micro and nanofiber formation.
- Melt electrospinning involves melting a polymer and shaping it into microfibers.
Compared to solution electrospinning, melt electrospinning has better reproducibility, better control, and does not require the toxic, volatile solvents used in solution electrospinning. Previous studies have found that the scaffolds’ chemical and physical properties can significantly affect stem cell differentiation. The researchers explored uncharted territory through an investigation into the effect of melt electrospun fiber diameter on scaffold shape and porosity. The researchers also studied the scaffolds’ capability to support stem cell growth and differentiation.
Methods
The research team custom built a melt electrospinning apparatus, which applied a high voltage to melted plastic granules to induce fiber formation. The researchers then varied different parameters of the electrospinning process (temperature, collecting distance, applied voltage, nozzle diameter) to observe the resulting effects on the diameter, porosity and strength of the fiber. These traits ultimately determine scaffold shape and topography. Additionally, the researchers cultured ESC-derived neural progenitors on the electronspun scaffolds to determine the scaffolds’ ability to support stem cell growth.
Important Findings
The researchers found that the fiber diameter is significantly affected by varying parameters of the electrospinning operation. Specifically, they determined that:
- Increasing the temperature of the melt increased the fiber diameter and spinning speed, which is key to shaping the scaffolds.
- Increasing the collecting distance increased fiber diameter.
- Increasing applied voltage decreased fiber diameter.
- Increasing the nozzle diameter increased fiber diameter, and decreasing nozzle diameter increased overlap in the fiber loops.
- Decreasing fiber diameter increased the porosity of the scaffold, leading to higher fiber strength and elasticity.
The researchers also found that ESC-derive neural progenitors adhered to the scaffolds well, and displayed clear neuronal differentiation due to the scaffolds’ physical cues.
To summarize, not only were the researchers able to produce scaffolds using melt electrospinning, they were able to consistently reproduce the desired fiber size distribution and scaffold topography. This experiment demonstrates that operational parameters in melt electrospinning can be adjusted to prevent fiber breakage or disorientation, major weaknesses of solution electrospinning. Furthermore, the researchers produced neuronal differentiation of ESCs using the electronspun scaffolds. The researchers’ combination of ESCs and melt electronspun fibers demonstrate an innovative way of producing malleable scaffolds for neural tissue engineering, without the use of toxic solvents.
Things to consider
Since scaffold porosity cannot be measured directly, the researchers combined experimental data with mathematical equations to determine the theoretical porosity of the scaffold as affected by different nozzle sizes. While the assumptions made in this stage of experiment are reasonable and well calculated, the final results may not be exact.
Additionally, the researchers specifically studied the fiber diameter’s impact on the scaffolds, and the operational parameters that affect the fiber diameter. However, the scaffolds’ properties are affected by many more factors, such as porosity of fiber mat, friction between fibers, thickness of fiber mat, and geometry. These factors were not evaluated, but they are important to the complete development of scaffolds in tissue engineering.
What does this mean for people living with SCI?
For the first time ever, this study demonstrated that electronspun microfibers can be used to support neuronal differentiation of ESCs. With more research and development, these scaffolds can become useful tools for tissue engineering applications, producing tissue products that can repair or replace damaged tissues found in people living with SCI.